Crop Production
Part of the Phosphorus Management series, this text details pros and cons of indices that can be used by soil and water professionals to determine and minimize the risk of phosphorus loss from a landscape.
Repeated application of excess phosphorus in the form of organic and inorganic fertilizers can cause phosphorus to build up in soil over time. When these soils erode and move along with rainwater during storm events and ultimately reach water bodies, water quality can be negatively impacted. One example is excessive growth of algae and aquatic weeds during summer months in water bodies or creeks.
Phosphorus loss from a landscape occurs in the form of both dissolved (water-soluble) and particulate (suspended soil particles) inorganic and organic phosphate. Dissolved phosphorus loss in runoff water from agricultural landscapes is one of the most difficult forms of pollution to control. The amount of phosphorus that can initiate water-quality issues is very low (0.08 to 0.1 parts per million). In the United States, freshwater eutrophication is estimated to cost $2.2 billion annually in lost services, and it takes decades to fix the problem through implementation of best management practices.
There are several indices that can be used to determine the risk of phosphorus loss from a landscape due to different management practices. Following is a general interpretation of the indices that are used to measure phosphorus loss risk from an environmental viewpoint.
Indices to Measure Phosphorus Loss Risk from Landscapes
Water Soluble Phosphorus
Water soluble phosphorus is a direct measure of soluble phosphorus in soil solution. It serves as an instantaneous indicator of plant-available phosphorus, phosphorus susceptible to runoff or leaching loss, or the amount of sorbed phosphorus that can be easily released from soil particles into the soil solution.
Comment: This is a quick and easy method used to determine labile phosphorus without use of any harsh chemicals. However, this method cannot be used for prediction of long-term phosphorus availability.
Soil Test Phosphorus
Soil test phosphorus uses extractants to measure soil phosphorus levels and is the most widely used tool within the United States for phosphorus fertilizer recommendation. It is used as an indicator of long-term phosphorus availability for agricultural purposes.
The most common extractants used for soil test phosphorus are Mehlich-1, Mehlich-3, Morgan, Olsen, and Bray. Mehlich-1 is the soil test extractant primarily used in Alabama for all soils except soils from the Black Belt region. Lancaster/Mississippi extractant is the official soil test extractant for the calcareous soils of the Blackland Prairies of Alabama.
Based on the amount of soil extractable phosphorus, Alabama soils can be categorized as very low, low, medium, high, very high, or extremely high in terms of phosphorus levels for fertilizer recommendation. Very low soils are those that yield less than 50 percent of their potential and require large applications of phosphorus fertilizer. Conversely, extremely high soils are those with phosphorus levels at least five times above the optimum. Further addition of phosphorus has no yield benefits and may contribute to pollution of ground and surface waters.
Soil test phosphorus currently is used mostly for agronomic purposes; however, it is also used as an input in the phosphorus index for assessing environmental phosphorus loss risk.
Comment: Soil test phosphorus is not a suitable indicator for predicting environmental phosphorus loss risk since it fails to account for the phosphorus retentive capacity of the soil and potential release of phosphorus into the soil solution. Thus a soil test phosphorus cannot predict whether a soil will act as a phosphorus sink (capable of retaining additional phosphorus) or phosphorus source (pose risk for phosphorus loss).
Phosphorus Sorption Capacity
Phosphorus sorption capacity is used to determine the ability of a soil to fix or retain phosphorus. Soils with greater phosphorus sorption capacity will have less phosphorus loss risk and vice versa.
Phosphorus loss from soils during runoff depends on the extent of phosphorus retention by soil components. In acidic soils, iron and aluminum oxides and hydroxides play a dominant role in phosphorus retention. In calcareous soils (pH > 7), calcium compounds fix the phosphorus. Three approaches are typically used to measure the phosphorus sorption capacity of the soils:
- Multipoint batch isotherm experiments. This is a traditional and more rigorous method to determine the phosphorus sorption capacity of soil. Comment: Determination of phosphorus sorption capacity using multipoint batch isotherm is time consuming and expensive. This method is used by commercial laboratories only on special request.
- Single-point isotherm. This approach also has been used to determine the phosphorus sorption capacity of soils. This method uses an upper-end phosphorus concentration of a multipoint isotherm to determine soil phosphorus fixation potential. Comment: Compared to multipoint isotherm measurements, single-point isotherm is less time consuming and provides a ballpark estimate of phosphorus sorption capacity. This method is also used by soil testing laboratories only on special request.
- Soil test method. Phosphorus sorption capacity of acid soils also can be obtained through determination of iron and aluminum concentrations using extractants, namely oxalate, Mehlich-1, or Mehlich-3. For acidic soils, acid ammonium oxalate (oxalate extractant) solution is the most widely used extractant to predict the phosphorus sorption capacity of the soil. However, oxalate extractant cannot be used for alkaline soils (pH > 7) since calcium (the major cation responsible for phosphorus sorption) forms precipitate at higher pH (pH > 7). Currently there is no single extractant that has been widely used for estimating the phosphorus sorption capacity of alkaline soils. Comment: Due to the diversity of soils between geographic regions, it is difficult to have a universal soil extractant that can help quantify the phosphorus sorption capacity.
Phosphorus Saturation Ratio
Phosphorus saturation ratio is another measure that can be used to predict the environmental risk of phosphorus loss. It is calculated as the ratio of phosphorus held (sorbed) by the soil to the phosphorus sorption capacity
PSR = Sorbed phosphorus ÷ PSC
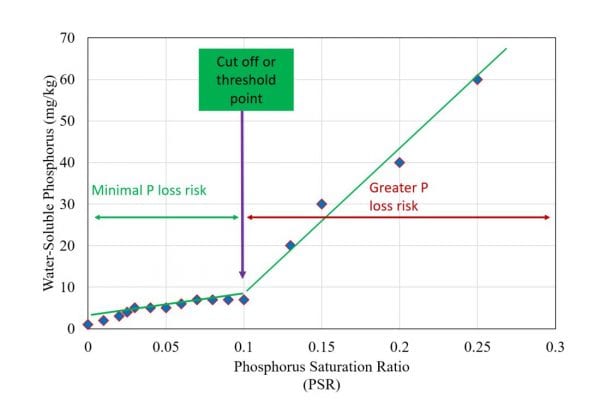
Figure 1. Relationship between water soluble phosphorus and phosphorus saturation ratio illustrating the concept of threshold phosphorus saturation ratio
The ratio of sorbed phosphorus to phosphorus sorption capacity is a useful tool to identify the “change point” at which a soil becomes a phosphorus source to the environment. When soils are extracted with water and plotted against the phosphorus saturation ratio, you get a change point, also referred to as the “threshold phosphorus saturation ratio” (figure 1). The change point may be defined as the point where the phosphorus concentration in the soil solution abruptly increases. Thus, the risk of phosphorus loss increases from any agricultural landscapes as the phosphorus saturation ratio reaches the threshold value.
Phosphorus saturation ratio for acid soils is typically derived using oxalate as the extractant (equation 1). However, phosphorus saturation ratio also can be derived from routine soil test extractants such as Mehlich-1 (equation 2) or Mehlich-3 (equation 3). Nair and co-workers1 have found this method effective for calculating phosphorus sorption ratio for sandy coastal plain soils of southeastern United States.
The following equations are used in calculating the phosphorus saturation ratio for acidic soils:
Equation 1
PSRox = Oxalate–P ÷ (Oxalate–Fe + Oxalate–Al)
Equation 2
PSRM1 = Mehlich-1–P ÷ (Mehlich-1–Fe + Mehlich-1–Al)
Equation 3
PSRM3 = Mehlich-3–P ÷ (Mehlich-3–Fe + Mehlich-3–Al)
Oxalate–P: oxalate-extractable phosphorus
Oxalate–Fe: oxalate-extractable iron
Oxalate–Al: oxalate-extractable aluminum
Mehlich-1–P: Mehlich-1-extractable phosphorus
Mehlich-1–Fe: Mehlich-1-extractable iron
Mehlich-1–Al: Mehlich-1-extractable aluminum
Mehlich-3–P: Mehlich-3-extractable phosphorus
Mehlich-3–Fe: Mehlich-3-extractable iron
Mehlich-3–Al: Mehlich-3-extractable aluminum
Here, phosphorus, iron, and aluminum are expressed in moles.
Comment: Although the phosphorus saturation ratio can be used as an indicator to determine phosphorus loss risk, like soil test phosphorus, this ratio fails to account for the true phosphorus retentive capacity of the soil. This limitation can be overcome by determination of soil phosphorus storage capacity.
Soil Phosphorus Storage Capacity
Soil phosphorus storage capacity can be effectively used as a tool to quantitatively predict the amount of phosphorus that can be added to a given mass or volume of soil before the soil becomes an environmental concern. Soil phosphorus storage capacity as proposed by Nair and Harris 2 depends on a threshold phosphorus saturation ratio and is typically determined using oxalate, since oxalate will extract most of the phosphorus held by iron and aluminum present in the soil. However, it can be potentially determined using common soil test extractants such as Mehlich-1 and Mehlich-3 by the development of conversion factors.
For simplicity, we have only discussed soil phosphorus storage capacity derived using the following oxalate extract equation:
SPSC (mg kg-1) = (Threshold PSROx – Soil PSROx) × (Ox-{Fe+Al}) × 31
Threshold PSROx: threshold phosphorus saturation ratio obtained from a population of soils from the same geographic regions and textural properties using oxalate as the extractant
Soil PSROx: phosphorus saturation ratio of individual soil samples derived using oxalate
Ox-Fe+Al: oxalate-extractable iron and aluminum expressed in moles
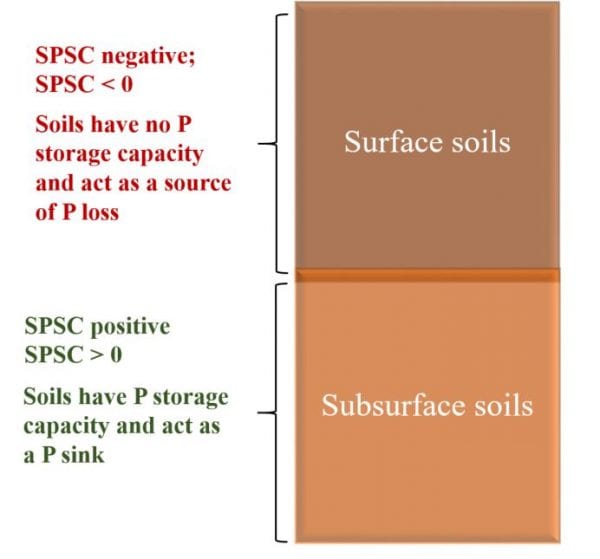
Figure 2. Soil phosphorus storage capacity for surface and subsurface horizons of a soil profile illustrating positive and negative soil phosphorus storage capacity.
Soil phosphorus storage capacity can be used as the capacity index of the soil and can predict whether the soil is acting as a phosphorus source or phosphorus sink. When soil phosphorus storage capacity is positive (SPSC > 0), the soil acts as a phosphorus sink, meaning it can retain phosphorus. When soil phosphorus storage capacity is negative (SPSC < 0), the soil acts as a phosphorus source. In figure 2 we assumed that the surface soils have no phosphorus retentive capacity due to phosphorus loading, resulting in negative soil phosphorus soil capacity, whereas the subsurface soils still have positive soil phosphorus storage capacity and can retain phosphorus.
Comment: Soil phosphorus storage capacity can be used to evaluate the amount of phosphorus that can be safely added to a soil before it becomes an environmental concern. This is important especially for fields with manure application history and where application rates are based on nitrogen requirements of the crop. Soil phosphorus storage capacity also can be used for predicting the remaining capacity of the soil if the phosphorus loading history is known.
References
1 Nair, V. D., K. M. Portier, D. A. Graetz, M. L. Walker. 2004. “An environmental threshold for degree of phosphorus saturation in sandy soils.” J. Environ. Qual. 33, 107–113. https://doi.org/10.2134/jeq2004.1070
2 Nair, V.D. and W. G. Harris. 2004. “A capacity factor as an alternative to soil test phosphorus in phosphorus risk assessment. New Zeal. J. Agric. Res. 47, 491–497. https://doi.org/10.1080/00288233.2004.9513616